While the Simpson Strong-Tie Tye Gilb R&D lab in Stockton is a large testing facility, the world’s largest R&D lab is Mother Nature herself. Natural disasters such as earthquakes or storms put our engineering designs to the test. In this week’s blog post, I’ll be turning attention to wall anchorage for out-of-plane forces and the lessons we have learned from Mother Nature so far.
The 1979 building code incorporated many of the lessons learned from the 1971 San Fernando earthquake. In 1994, Mother Nature put the 1979 building code to the test with the January 17 Northridge earthquake. The Northridge earthquake showed that some of the increased design and detailing requirements in the 1979 building code worked well to improve performance over what was observed in 1971. However, it also revealed to researchers that acceleration at the roof level of single story warehouse buildings were three to four times the ground acceleration. The combination of higher than expected acceleration and excessive deformation of the wall anchorage assembly caused many wall anchorage failures.
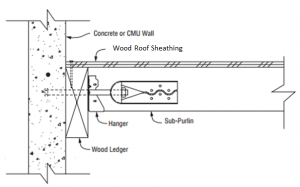
Several changes in the design forces used for wall anchorage and additional detailing requirements were incorporated in the 1997 Uniform Building Code. The requirements have been refined with each new building code, but overall the requirements and design forces have remained about the same under the current International Building Code. Wall anchorage design is governed by ASCE 7-05 and ASCE 7-10 Section 12.11. These provisions aim to mitigate the brittle wall anchorage failures observed in past earthquakes by increasing the design force and in Seismic Design Categories C through F, requiring:
- continuous ties,
- limiting the maximum sub-diaphragm aspect ratio,
- increasing the design force for the steel elements of the wall anchorage system,
- precluding the use of toenails or nails in withdrawal or cross-grain bending or cross-grain tension,
- embedded straps be attached or hooked around reinforcing steel,
- considering effects of eccentricity on system,
- considering additional wall anchorage force at pilasters.
A material overstrength factor of 1.4 is used for the steel elements of the anchorage system to ensure sufficient nominal overstrength for the entire wall anchorage system. This 1.4 factor applies to all the steel components in the wall anchorage system, including the sub-diaphragms and continuity ties. In addition, edge nailing of the roof or floor sheathing to a wood framing member may not be considered to provide out-of-plane capacity. Also note that the wall anchorage design equations for wall anchorage have been updated in ASCE 7-10 to account for the span length of diaphragms. This may help reduce design forces where diaphragm spans (defined by distance between walls) are less than 100 feet.
It’s worthwhile to point out 2009 & 2012 IBC Section 1908.1.9 recognized ACI 318 Section D.3.3.4 and D.3.3.5 ductility requirements for concrete anchorage should not be applied on top of the ASCE 7 wall anchorage system requirements. Since the ASCE anchorage design forces have already been factored to protect against brittle failure, applying the additional ductility requirements from ACI 318 Appendix D was overly conservative.
The 1999 SEAOC Blue Book by the 1999 Seismology Committee and the September 2008 SEAOC Blue Book Tilt-up Buildings discusses much of the philosophy for the current code wall anchorage design provisions. In addition, Simpson Strong-Tie has a panelized roof system Technical Bulletin (T-PRS12) with several wall anchorage design examples and details. Woodworks also provide resources and case studies of panelized roof systems. Some building jurisdictions have their own requirements in addition to those in the IBC, such as the City of Los Angeles. The designer should check with the Building Department with jurisdiction over the project to determine if they have any additional wall anchorage design requirements.
What lessons do you expect Mother Nature to reveal to the industry in the next “Big One”? Let me know in the comments.
– Paul
What are your thoughts? Visit the blog and leave a comment!
I am a big fan of this publication, and I’m glad you updated it.
Tom
Hurricane Sandy, will there ever be required design
for water forces for anchorage of houses to foundations for coastal housing? It
appears that a lot of homes where just washed off their foundation. I realize
some had their foundations eroded out from under them but it looks like some
were just floated or pushed off the foundation by water forces. I know FEMA has
their own requirements for houses and commercial buildings within the flood
plain, and I know there is some information on coastal zones but I am not
familiar with any specific design requirements for what we saw as results from
Sandy. What else do others see that we
will learn from Sandy?
Evan
FEMA flood requirements vary based on the type of flooding. For areas where floodwater is not expected to be moving, you can use floodproofing or floodventing to allow the water to enter and exit an area under the house. In areas where there is expected to be moving water, called a V Zone, buildings are required to be elevated above the level of a 100 year flood. The flood loads are so high that buildings are not expected to be able to resist them, so you basically avoid them. Enclosures on the ground level in V Zones are limited in size and walls must be designed to break away under flood loads to prevent transferring forces to the building and foundation.
Following Hurricane Katrina, the flood elevations in some areas of the Gulf Coast were raised by 10-feet or more. It is possible that the flood requirements in some areas affected by Sandy will be similarly changed, which would require elevated construction. A lot of New Jersey and New York coastal construction probably predates FEMA flood requirements, but structures damaged over 50% will have to be rebuilt to the new regulations.
Paul;
Thanks for getting back to me. I was aware of the flood requirements for buildings as you stated and in fact we did a commercial building where we could not get the building above the flood plain. It was a building that was built in the early 1900’s and burned beyond repair so this was a replacement building in a town where the main street is below flood plain and the building was built between 2 other buildings and tight to the sidewalks. So we were locked into the floor elevation. So we built the foundation to resist the V Zone forces which basically we ended up with 2 ft wide foundation/stem walls with lots of rebar that extended 12” above the flood plain elevation. Then at the openings we put slits in the ends of the concrete wall sections and there were flood panels built to go in them which are stored out of the way and manually installed during predicted flood events. Which because of our location we do not have situations where we do not have forewarning of the events. This building was a 3 story wood framed building with a CMU brace panel in 2 walls and steel moment frames on the other 2 walls and has brick veneer on the exposed walls. It was actually a very unique building structurally. It had a fair bit of Simpson products in it.
I agree about the construction predating the FEMA regulations as almost all of our down town district in our little town as well as a number of other surrounding towns are built below the flood elevations set by FEMA. Most of our maps first went into effect in May, 1980.
We also did an entire row of duplexes with garages on the bottom floor with all concrete walls and did the flood vents. The foundations were poured directly on what we call blue rock which is so hard a hoe mounted rock breaker really does not tough it. We also doweled the footings into the rock.
Also you mentioned the 50% damage threshold for rebuilding and I have heard it a number of time but out here the local and state regulators say that that portion of the building even if less than 50% has to be built back to the new requirements which meant in one project this summer we had a barn partially burn down (less than 50%) and they said that the floor in the area where we had to replace walls and roof had to have a raised floor which you can imagine was not feasible in a barn, it is currently just sitting there with part of it gone which is not very stable with winds. I recently called FEMA on this and they said they knew of that also but they could not direct me to any document that would back that up. Just out of curiosity do you know of where this is stated in the FEMA documents?
The thing that you shared that was news to me (as we are out here on the west coast) is that they raised the flood elevation as much as 10 ft. That was a wise move on FEMA’s part but I bet it hit with a lot of resistance by the owners that lost their homes.
Evan