We know it’s tough going to school and majoring in structural engineering or architecture. You probably weren’t aware of this, but I went to Brooklyn Technical High School and we were required to take mechanical drafting, electrical engineering and wood/metal shop before we selected majors at the end of our sophomore year. I actively avoided majoring in architecture and engineering because, while I was a whiz at the lathe in metal shop, I was much less talented in some of the other engineering subjects.
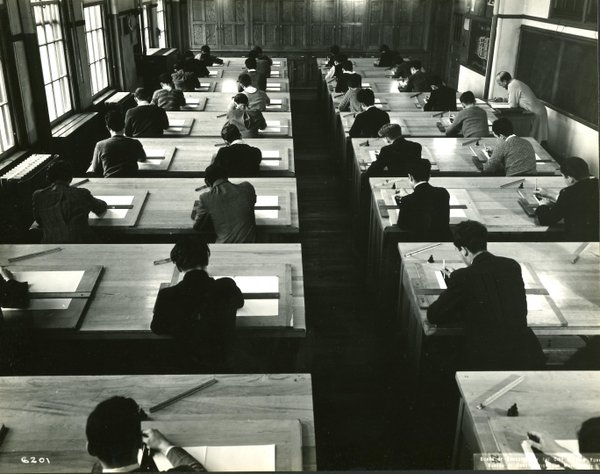
I sometimes wish I had been better at them, because getting a degree in structural engineering and architecture isn’t just cool (where else can you get college credit or money to break stuff?), it can help you improve the lives of others and even make them safer. Simpson Strong-Tie Company, Inc. established the structural engineering/architecture scholarship program to assist architecture and structural engineering students by supporting their education and encourage them to design and build safer structures in their local communities.
And it seems as though there are more and more students committed to those goals, too. Last year, Simpson Strong-Tie awarded 49 scholarships of $2,000. The year before, Simpson Strong-Tie awarded 38 scholarships of $1,000. This year, Simpson Strong-Tie is offering up to 67 scholarship awards of $2,000 for the 2016/2017 academic school year. Applicants must be enrolled as juniors or seniors in full-time undergraduate study (60 semester hours or equivalent) majoring in architecture, structural engineering or construction management at the following colleges or universities for the entire upcoming academic year:
- Arizona State University
- Boise State University
- Brigham Young University
- California State Polytechnic University, Pomona
- California State Polytechnic University, San Luis Obispo
- California State University, Fresno
- California State University, Fullerton
- California State University, Long Beach
- California State University, Sacramento
- Clemson University
- Florida International University
- Georgia Institute of Technology
- Iowa State University
- Louisiana State University
- Milwaukee School of Engineering
- NYU Polytechnic School of Engineering
- North Carolina State University
- Ohio State University, Columbus
- Oklahoma State University
- Oregon Institute of Technology
- Oregon State University
- Penn State University Park
- Portland State University
- Purdue University, West Lafayette
- Southern California Institute of Architecture
- Texas Tech University
- University of Arizona
- University of California, Berkeley
- University of California, Davis
- University of California, Irvine
- University of California, Los Angeles
- University of California, San Diego
- University of Cincinnati
- University of Florida
- University of Idaho
- University of Illinois at Urbana-Champaign
- University of Miami
- University of Michigan
- University of Nevada, Las Vegas
- University of North Texas
- University of Southern California
- University of Texas, Arlington
- University of Texas, Austin
- University of Washington
- University of Wyoming
- Virginia Polytechnic Institute and State University
- Washington State University
The scholarship application will be available on the Simpson Strong-Tie website as of March 15, so if you know any students enrolled in a structural engineering, architecture or construction management major at the schools listed above, you should advise them about this wonderful opportunity. If you have any questions or comments, please let us know in the comments below.