Back in the year 2000, the U.S. Department of Defense (DoD) was charged with incorporating antiterrorism protective features into the planning, design and execution of its facilities. The main document developed to meet this requirement is the Unified Facilities Criteria “DoD Minimum Antiterrorism Standards for Buildings” (UFC 4-010-01). The current version was published in October 2013. This document covers what is most commonly referred to as “blast” design.Continue Reading
Construction Referees: Evaluation Processes for Alternative Building Products
There are products used in every building not referenced by the codes or standards.
These products can impact safety, public health and general welfare through their effect on structural strength, stability, fire resistance and other building performance attributes. I-code Section 104.11 (Alternative materials, design, and methods of construction and equipment) provides guidance on how these products are approved for use in the built environment and identifies the Building Official as the decision-maker. This is similar to a referee determining a player’s compliance with the rules.
Building Officials see submittals for a wide variety of alternative building products ranging from the simple to the very complex. The amount of data included in these submittals and their relevance and completeness varies significantly from insufficient and minimal to complete and very thorough. In the absence of publicly developed and majority-approved provisions, the Building Official is tasked to ensure the data provided is appropriate and adequately proves the alternative product meets code intent to protect public safety, no matter the product type or complexity. This is compared to the robust code and standards process in which committees with balanced representation publicly develop and deliberate on provisions in order to protect public safety. The question arises whether the 104.11 requirement implies that a similar robust process be used in the development of test and evaluation requirements for alternative products as is used for the development of code and standard provisions where there is public debate, resolution of negative opinions and a majority approval of the requirements. Requiring a similar code development process for alternative products would seems to make sense. Otherwise, a less rigorous process might be employed by those seeking to avoid a more robust code and standard process so as to achieve quicker and less stringent approval for their alternative products.
Some may argue that having to use a “code-like” evaluation process for alternative products would add too much of a burden in time and cost, and that it’s not necessary since individual registered design professionals and building officials have enough time, resources and expertise to determine acceptability. But this begs the question of why a similar public majority-approval process should not be required for new products as it is required for code-referenced products. Another question that comes up is ongoing acceptance of an alternative product, as their manufacture may have changed since their approval. Additionally, different jurisdictions have different expertise and resources and this can lead to different standards for approval for alternative products, leading to inconsistency.
Is there a solution which balances providing innovative and cost-effective alternative building product solutions to the industry in a timely manner with providing a thorough product assessment using a process similar to the codes and standards to better ensure consistency and public safety? Accredited building product certification companies, or evaluation service companies, that use a publicly developed and majority-approved acceptance or evaluation criteria and publish an evaluation report with the product’s description, design and installation requirements and limitations provide such a solution. These evaluation service companies are a third-party resource for building officials to assist in their determination of whether an alternative product meets code intent and should be approved for use in their jurisdiction.
The number of evaluation service companies has been increasing. The ICC Evaluation Service and the IAPMO Uniform Evaluation Service, two of the better-known such companies, are both ANSI accredited to ISO/IEC 17065 (Conformity Assessment – Requirements for bodies certifying products, processes, and services) to provide building-code product certifications (ICC-ES, IAPMO UES). However, accreditation by itself mainly verifies a certain process is implemented to ensure consistency and confidentiality. Both companies also have a public acceptance or evaluation criteria process. This process includes an evaluation committee made up of building enforcement officials. These officials evaluate the proposed criteria, listen to expert and industry input and only approve the criteria by a majority vote if products evaluated to those criteria will meet code intent. This is similar to how the codes and standards are developed — a transparent public process and a majority approval of requirements and not just an opinion of one or a couple of individuals.
The alternative building product review process for ICC-ES and IAPMO UES is similar and has the following important components.
- CRITERIA: The accredited product evaluation service develops an acceptance or evaluation criteria, with the manufacturer’s and public’s input, that is publicly debated, revised and ultimately approved by a majority vote of a committee of building enforcement officials.
- TESTING: The manufacturer contracts out to an accredited independent third-party test laboratory to either perform or witness the product testing in accordance with the criteria.
- REVIEW: Registered design professionals with the accredited product evaluation service evaluate the testing and analyses performed and sealed by registered design professionals with the manufacturers or their representatives. The product evaluation service then publishes the evaluation report to their website, and the report typically contains the product description, design and installation requirements and
limitations.
- CONTINUOUS COMPLIANCE: The manufacturer’s quality system is inspected at least annually by the product evaluation service or an accredited third-party
inspection agency to ensure that the product currently being manufactured is the same as that which was evaluated.
While the term “product evaluation” is sometimes used, it is often “product certification” or “product conformity assessment.” ISO/IEC Guide 2:2004 defines “conformity assessment” as “Any activity concerned with determining directly or indirectly that relevant requirements are fulfilled. Some “product certification” companies also provide “product listing” services for when testing and evaluation requirements for the product are already in code-referenced consensus standards, making the development of acceptance criteria unnecessary, thus simplifying the process.
A couple of previous blog posts on evaluation or code reports that you may find informative discuss steps to obtain an evaluation or code report and provide a checklist to determine adequacy of a report.
A mechanism is available to the building industry to provide innovative and cost-effective alternative building products in a timely manner that implements a public and majority product acceptance criteria process, similar to the codes and standards development process. This solution involves the Building Official referencing building product evaluation service reports, based on acceptance criteria, offering a robust evaluation better ensuring that an alternative product meets code intent, thus protecting the public. In fact, several jurisdictions do require evaluation service reports for alternative products.
Should there be an easier path to approval for alternative products than for code-referenced products? What is a reasonable path to product approval? What basis do you use in reviewing evaluation or code reports to determine whether an alternative product is “in or out of -bounds”? We’d love to hear your thoughts.
Five Simpson Strong-Tie employees had the opportunity to participate in a week-long Habitat for Humanity build in the small town of Amarante, Portugal, in late April. The company decided to allocate the funds for the CWP to Habitat’s Global Village program, allowing these employees to help renovate and remodel the older home of a widowed mother (Doña Margarida Ribiero) and daughter (Sonia) living in the Portuguese countryside.
Building with Habitat for Humanity in Portugal
Five Simpson Strong-Tie employees had the opportunity to participate in a week-long Habitat for Humanity build in the small town of Amarante, Portugal, in late April. The group was originally scheduled to work on a Habitat project in Nepal late last year as part of Habitat’s Jimmy and Rosalynn Carter Work Project (CWP), but following the signing of a new constitution and civil unrest in the country, the project was canceled.Continue Reading
Treated Lumber and Trusses (and the One Condition Under Which MPC Wood Trusses Shouldn’t Be Used)
What do a chicken house, a water treatment plant and a raised wood floor system all have in common? Very likely, they all involve preservative-treated lumber. They’re also all examples of common environments in which preservative-treated, metal-plate- connected (MPC) wood trusses may be specified.
Although trusses are successfully used in a variety of environments that require treated lumber, the first mention of “treated lumber” usually sends up a red flag in a truss design office. While the corrosion protection of truss plates is no different from the corrosion protection of any other steel fastener or hanger that comes in contact with treated lumber, there are a few more considerations that come into play whenever treated lumber is going to be used in a truss application.
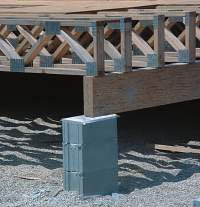
When fire-retardant-treated lumber or preservative-treated lumber is specified, the first (and easiest) step is to determine whether standard G60 truss plates are acceptable for use with the treated lumber, or whether the chemical treatment requires additional protection of the plates. Recent blog posts have discussed how fasteners are evaluated for corrosion resistance and how the Corrosion Resistance Classifications in our catalog help facilitate selection of hardware and fasteners for different types of treated wood and environmental conditions. Similar guidelines are also available for determining the proper metal connector plate for different wood treatments. For example, when using the sodium borate–based preservatives and fire retardants, standard G60 galvanized metal connector plates are acceptable. However, ammoniacal/alkaline/amine copper quaternary preservative types require more protection, such as G185, ASTM A153 galvanized- or stainless-steel truss plates. The complete guidelines – Quick Guide for Alternative Preservative Treatments with Metal Connector Plates – are available from the SBCA website.
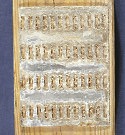
When trusses are used in particularly corrosive environments such as coastal environments or salt storage buildings, the ANSI/TPI 1 standard lists coatings that will provide increased corrosion protection for the plates (see insert, below).
The paint coating systems listed in (a) and (b) have been specified in the TPI standard since 1985. These paint coatings, which are applied to the truss plates after the trusses are manufactured, provide alternatives to the double-dipped galvanized or stainless-steel plates used in coastal high hazard areas. In fact, the ANSI/TPI 1 Commentary states that one study – SSPC Report 87-08, Evaluation of Coatings for Metal Connector Plates – concluded that the paint coating systems over standard galvanized plates would be expected to outperform the double-galvanized metal connector plates in field use.
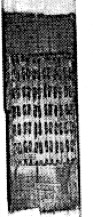
Once the necessary corrosion protection of the plates has been addressed, the next consideration is the effect of certain lumber treatments on the truss plates’ lateral resistance, or tooth-holding capacity. Fire-retardant treatments generally require strength reductions to be applied to both the lumber and metal connector plate design values. The proprietary treatment manufacturer specifies these design reductions. As soon as the specific treatment is known, the appropriate design reductions can be easily applied by the truss design software and noted on the truss design drawing accordingly.
Besides lumber treatment, there may be other reasons for plate design reductions whenever extra galvanization or special coatings are required. While extra galvanization itself does not necessarily require a reduction in plate values, if the treated lumber’s moisture content (MC) exceeds 19% at the time of truss fabrication, then a 20% reduction to the tooth-holding values is required. The same 20% reduction applies if the environment for the intended end use of the trusses is expected to result in wood moisture content exceeding 19%.
Special Considerations and Red Flags
One corrosive environment that requires special consideration is an enclosed swimming pool. ANSI/TPI 1 requires that trusses be separated from the pool environment by a vapor barrier and be separately ventilated from the pool environment. The exception to this requirement is if the truss plates are made with a stainless steel that is not susceptible to stress corrosion cracking (SCC), i.e., not Types 304 and 316. Since truss plates made with SCC-resistant stainless steel are not readily available (if at all), a vapor barrier is basically required anytime trusses are used over enclosed swimming pools.
Another important consideration in roof truss applications involving treated lumber is the effect of elevated temperatures. For example, when FRT lumber is going to be used in an environment where high moisture content will exist, an FRT formulated for exterior use may be specified. However, if the exterior FRT has not been tested with elevated temperatures as specified in TPI 1 Section 6.4.9.1, it should not be used in a roof application.
But the biggest concern when treated lumber is specified for use in metal-plate-connected wood trusses has nothing to do with corrosion at all. When a truss Designer gets a job that calls for a preservative treatment for exterior use or an exterior FRT, the very first question will be why is an exterior treatment required/what is the application? Although trusses can be adequately designed for many types of environments, there is one environment that does not mix well with metal-plate- connected wood trusses – exposed exterior applications. The TPI/WTCA Guidelines for Use of Alternative Preservative Treatments with Metal Connector Plates concludes with the following statement:
When trusses are exposed to repeated wetting and drying, the corresponding swelling/shrinkage of the wood causes what is commonly referred to as truss plate “back out”. Since the ability of a truss plate to provide lateral resistance depends on the teeth having adequate embedment into the wood members, any plate “back out” or withdrawal from the lumber due to weathering has an adverse effect on the load capacity of the truss plate.
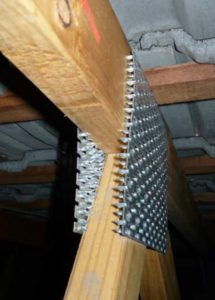
For this reason, MPC wood trusses must be protected from the elements, from the time they are built and stored through the extent of their life in service. High moisture content that is consistently high can be accounted for; but if the trusses will be exposed to moisture cycling, then it is time to consider something other than a metal-plate-connected wood truss.
What are your experiences with treated lumber and/or corrosive environments and wood trusses? Let us know in the comments section below.
Simpson Strong-Tie® Research and Testing Lab Grand Opening, WSU Pullman
On Thursday, May 5, 2016, Washington State University at Pullman, state dignitaries, construction leaders, WSU construction alumni, PACCAR management, Simpson Strong-Tie management and the press celebrated the grand opening and dedication of the PACCAR Environmental Technology Building (PETB) and the Simpson Strong-Tie Research and Testing Laboratory.
The Simpson Strong-Tie team comprised senior leadership, engineering and marketing representatives, led by our CEO, Karen Colonias. In her speech at the opening ceremony, Karen Colonias highlighted the leadership of Simpson Strong-Tie in the engineering and construction materials industry in the U.S. and the world. She emphasized the longstanding partnership between WSU and Simpson Strong-Tie, which spans over twenty years of collaboration in various testing and code development programs, and communicated our excitement at the opportunity to collaborate more closely with WSU’s highly respected engineering department on testing and engineering programs.
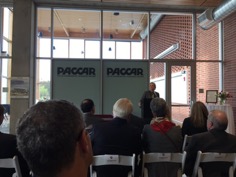
The Paccar Environmental Technology Building (PETB) is 96,000 square feet and houses the Composite Materials and Engineering Center (CMEC) – a highly integrated hub of interdisciplinary research and education in the areas of renewable materials, sustainable design, water quality, and atmospheric research. The shared space in this new building will foster the synergy needed to find new solutions to complex industry problems, such as creating human environments that are at once safe, economical and resilient.
The Simpson Strong-Tie® Research and Testing Lab at Washington State University (WSU) is a versatile laboratory designed specifically for the structural testing and prototyping of tall timber buildings, post frame buildings, concrete durability, building repair and retrofit and deck safety, as well as seismic and wind mitigation.
The lab includes a high-capacity reaction 28′ x 46′ concrete floor area with tie-downs, 75-kip capacity at two foot centers through the floor area; a high-capacity wall 28′ long by 2’thick by 18′ tall strong wall that is capable of withstanding a 200-kip reaction in any direction; a central 90-gallon-per-minute hydraulic pump, overhead crate and concrete mixing station. The laboratory is a dynamic space to test new material and design concepts developed in the PETB. This is one of the most visible spaces in the PETB and includes capabilities for mock-ups of new building systems, structural testing and advanced digital manufacturing. Adjoining the lab is an outdoor 32′ by 52′ reaction slab that allows for project display (e.g., Solar Decathlon competition), for developing taller and or larger structures than would be possible on the interior strong floor and for natural weather exposure testing.
The lab is part of the Composite Materials and Engineering Center (CMEC), which has been a leader in the development of wood composite materials for more than 65 years. It is an International Code Council–accredited testing facility. The laboratory highlights engineered wood composites and is constructed of cross-laminated timber, glulam, Parallam and, of course, Simpson Strong-Tie® No- Equal connectors.
Simpson Strong-Tie and WSU, as Karen Colonias mentioned in her speech, have a longstanding and productive partnership going back over 20 years. The two institutions have worked together in a number of areas, including new product testing, deck safety and seismic risk mitigation.
This year, Simpson Strong-Tie made a significant commitment and established the Simpson Strong-Tie Excellence Fund at the Voiland College of Engineering and Architecture at Washington State University (WSU). The fund provides an annual gift of $100,000 per year over the next eight years to support the new Simpson Strong-Tie® Research and Testing Lab in the PACCAR Environmental Technology Building (PETB). In addition to the lab, the Excellence Fund will support fellowships for professors and graduate students to present research findings, brainstorm about future research and conduct continuing education training.
The faculty of the Composite Materials and Engineering Center is committed to addressing the challenge of restoring and improving the U.S. civil infrastructure and offering an integrated approach linking material discovery, manufacturing innovation, product development, and customized design methodologies that will lead to high-performing, cost-effective solutions for the built environment. The core faculty possess diverse expertise that spans materials science (polymers, wood, cement, steel), durability and corrosion protection, manufacturing and sustainable design. The faculty also has a long history of involvement in developing building codes, standards and product acceptance criteria.
This year, the WSU Voiland College of Engineering and Architecture has more than 1,050 students enrolled in civil engineering, architecture and construction management programs. The alumni from these programs are founders of and senior executives in America’s top construction and design firms. The Wall Street Journal ranked WSU among the 25 universities whose graduates are top-rated by industry recruiters, and the Civil Engineering program is the 13th largest in the nation.
On October 29, 2016, and in line with this partnership, Simpson Strong-Tie is conducting its first annual engineering symposium at Washington State University Pullman. In this symposium, Simpson Strong-Tie engineers will share with the engineering and construction management students the various career opportunities that are available in the industry upon their graduation and introduce them to the exciting history of research and innovation at Simpson Strong-Tie. The Symposium will also include testing in the new lab of our No-Equal structural connectors and solutions.
At Simpson Strong-Tie, we are excited to be strengthening the partnership and increasing the collaboration with WSU faculty and students. We are looking forward to an extended and outstanding relationship that drives research and innovations and introduces new methods to design and construct safer, more resilient, sustainable and economical structures.
Onward and Upward!
Louay Shamroukh, P.E., S.E.
Engineering Manager, Northwestern U.S.
Simpson Strong-Tie® Strong-Wall® Wood Shearwall – The Latest in Our Prefabricated Shearwall Panel Line Part 2
In last week’s blog post, we introduced the Simpson Strong-Tie® Strong-Wall® Wood Shearwall. Let’s now take a step back and understand how we evaluate a prefabricated shear panel to begin with.
First, we start with the International Building Code (IBC) or applicable state or regional building code. We would be directed to ASCE7 to determine wind and seismic design requirements as applicable. In particular, this would entail determination of the seismic design coefficients, including the response modification factor, R, overstrength factor, Ωo, and deflection amplification factor, Cd, for the applicable seismic-force-resisting system. Then back to the IBC for the applicable building material: Chapter 23 covers Wood. Here, we would be referred to AWC’s Special Design Provisions for Wind and Seismic (SDPWS) if we’re designing a lateral-force-resisting system to resist wind and seismic forces using traditional site-built methods.Continue Reading
Hurricane Strong
This week will see the ultimate combination of events intended to raise public awareness of the necessity for disaster-resistant construction: It is week three of ICC’s Building Safety Month; National Hurricane Preparedness Week, as proclaimed by the U.S. president; the NOAA Hurricane Awareness Tour of the Gulf Coast; and the kickoff of the new HurricaneStrong program.
The ICC says that “Building Safety Month is a public awareness campaign to help individuals, families and businesses understand what it takes to create safe and sustainable structures. The campaign reinforces the need for adoption of modern, model building codes, a strong and efficient system of code enforcement and a well-trained, professional workforce to maintain the system.” Building Safety Month has a different focus each week for four weeks. Week One is “Building Solutions for All Ages.” Week Two is “The Science Behind the Codes.” Week Three is “Learn from the Past, Build for Tomorrow.” Finally, Week Four is “Building Codes, A Smart Investment.” Simpson Strong-Tie is proud to be a major sponsor of Week Three of Building Safety Month.
National Hurricane Preparedness Week is recognized each year to raise awareness of the threat posed to Americans by hurricanes. A Presidential Proclamation urged Americans to visit www.Ready.gov and www.Hurricanes.gov/prepare to learn ways to prepare for dangerous hurricanes before they strike. Each day of the week has a different theme. The themes are:
⦁ Determine your risk; develop an evacuation plan
⦁ Secure an insurance check-up; assemble disaster supplies
⦁ Strengthen your home
⦁ Identify your trusted sources of information for a hurricane event
⦁ Complete your written hurricane plan.
This week also marks the NOAA Hurricane Awareness Tour, where NOAA hurricane experts will fly with two of their hurricane research aircraft to five Gulf Coast Cities. Members of the public are invited to come tour the planes and meet the Hurricane Center staff along with representatives of partner agencies. The goal of the tour is to raise awareness about the importance of preparing for the upcoming hurricane season. The aircraft on the tour are an Air Force WC-130J and a NOAA G-IV. These “hurricane hunters” are flown in and around hurricanes to gather data that aids in forecasting the future of the storm. As with Hurricane Preparedness Week, each day of the tour features a different theme. Simpson Strong-Tie is pleased to be a sponsor for Thursday, when the theme is Strengthen Your Home. Representatives from Simpson Strong-Tie will be attending the event on Thursday to help educate homeowners on ways to make their homes safer.
Finally, this week is the official kickoff of a new hurricane resilience initiative, HurricaneStrong. Organized by FLASH, the Federal Alliance for Safe Homes and in partnership with FEMA, NOAA and other partners, the program aims to increase safety and reduce economic losses through collaboration with the most recognized public and private organizations in the disaster safety movement. HurricaneStrong is intended to become an annual effort, with activities starting prior to hurricane season and continuing through the end of the hurricane season on November 30. To learn more, visit www.hurricanestrong.org.
Experts consider these public education efforts to be more important every year, as it becomes longer since landfall of a major hurricane and as more and more people move to coastal areas. The public complacency bred from a lull in major storms has even been given a name: Hurricane Amnesia.
All these efforts may be coming at a good time, assuming one of the hurricane season forecasts is correct. A forecast from North Carolina State predicts an above-average Atlantic Basin hurricane season. On the other hand, forecasters at the Department of Atmospheric Science at Colorado State University are predicting an approximately average year.
Are you prepared for the natural hazards to which your geographic area is vulnerable? If not, do you know where to get the information you need?
Temblor Insights: Is the San Andreas “locked, loaded, and ready to go?”
Editor’s Note: This is a republished blog post with an introduction by Jeff Ellis.
This is definitely an attention-grabbing headline! At the National Earthquake Conference in Long Beach on May 4, 2016, Dr. Thomas Jordan of the Southern California Earthquake Center gave a talk which ended with a summary statement that the San Andreas Fault is “locked, loaded and ready to go.”
The LA Times and other publications have followed up with articles based on that statement. Temblor is a mobile-friendly web app recently developed to inform homeowners of the likelihood of seismic shaking and damage based on their location and home construction. The app’s creators also offer a blog that provides insights into earthquakes and have writtene a post titled “Is the San Andreas ‘locked, loaded, and ready to go’?” This blog post delves a bit deeper to ascertain whether the San Andreas may indeed be poised for the “next great quake” and is certainly a compelling read. Drop, cover and hold on!
Volkan and I presented and exhibited Temblor at the National Earthquake Conference in Long Beach last week. Prof. Thomas Jordan, USC University Professor, William M. Keck Foundation Chair in Geological Sciences, and Director of the Southern California Earthquake Center (SCEC), gave the keynote address. Tom has not only led SCEC through fifteen years of sustained growth and achievement, but he’s also launched countless initiatives critical to earthquake science, such as the Uniform California Earthquake Rupture Forecasts (UCERF), and the international Collaboratory for Scientific Earthquake Predictability (CSEP), a rigorous independent protocol for testing earthquake forecasts and prediction hypotheses.
In his speech, Tom argued that to understand the full range and likelihood of future earthquakes and their associated shaking, we must make thousands if not millions of 3D simulations. To do this we need to use the next generation of super-computers—because the current generation is too slow! The shaking can be dramatically amplified in sedimentary basins and when seismic waves bounce off deep layers, features absent or muted in current methods. This matters, because these probabilistic hazard assessments form the basis for building construction codes, mandatory retrofit ordinances, and quake insurance premiums. The recent Uniform California Earthquake Rupture Forecast Ver. 3 (Field et al., 2014) makes some strides in this direction. And coming on strong are earthquake simulators such as RSQsim (Dieterich and Richards-Dinger, 2010) that generate thousands of ruptures from a set of physical laws rather than assumed slip and rupture propagation. Equally important are CyberShake models (Graves et al., 2011) of individual scenario earthquakes with realistic basins and layers.
But what really caught the attention of the media—and the public—was just one slide
Tom closed by making the argument that the San Andreas is, in his words, “locked, loaded, and ready to go.” That got our attention. And he made this case by showing one slide. Here it is, photographed by the LA Times and included in a Times article by Rong-Gong Lin II that quickly went viral.
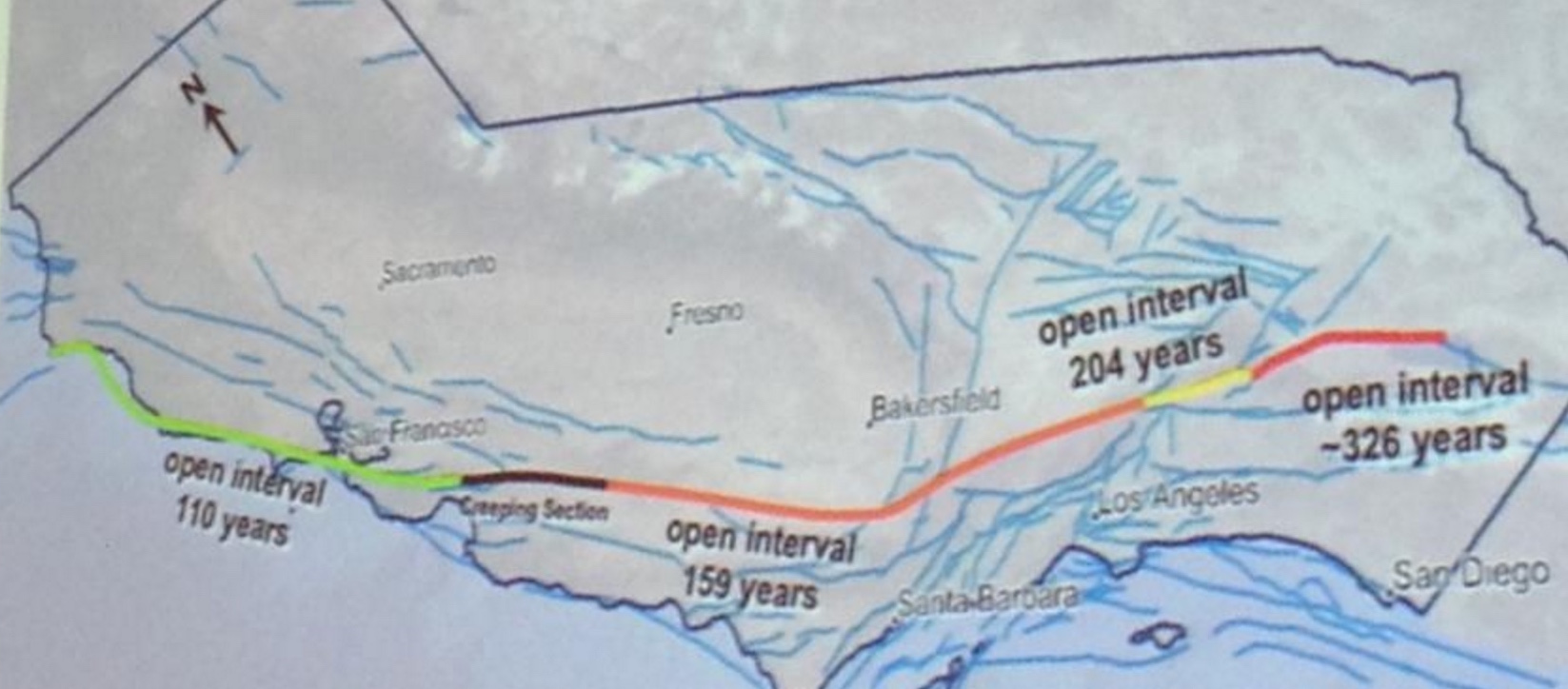
Believe it or not, Tom was not suggesting there is a gun pointed at our heads. ’Locked’ in seismic parlance means a fault is not freely slipping; ‘loaded’ means that sufficient stress has been reached to overcome the friction that keeps it locked. Tom argued that the San Andreas system accommodates 50 mm/yr (2 in/yr) of plate motion, and so with about 5 m (16 ft) of average slip in great quakes, the fault should produce about one such event a century. Despite that, the time since the last great quake (“open intervals” in the slide) along the 1,000 km-long (600 mi) fault are all longer, and one is three times longer. This is what he means by “ready to go.” Of course, a Mw=7.7 San Andreas event did strike a little over a century ago in 1906, but Tom seemed to be arguing that we should get one quake per century along every section, or at least on the San Andreas.
Could it be this simple?
Now, if things were so obvious, we wouldn’t need supercomputers to forecast quakes. In a sense, Tom’s wake-up call contradicted—or at least short-circuited—the case he so eloquently made in the body of his talk for building a vast inventory of plausible quakes in order to divine the future. But putting that aside, is he right about the San Andreas being ready to go?
Because many misaligned, discontinuous, and bent faults accommodate the broad North America-Pacific plate boundary, the slip rate of the San Andreas is generally about half of the plate rate. Where the San Andreas is isolated and parallel to the plate motion, its slip rate is about 2/3 the plate rate, or 34 mm/yr, but where there are nearby parallel faults, such as the Hayward fault in the Bay Area or the San Jacinto in SoCal, its rate drops to about 1/3 the plate rate, or 17 mm/yr. This means that the time needed to store enough stress to trigger the next quake should not—and perhaps cannot—be uniform. So, here’s how things look to me:
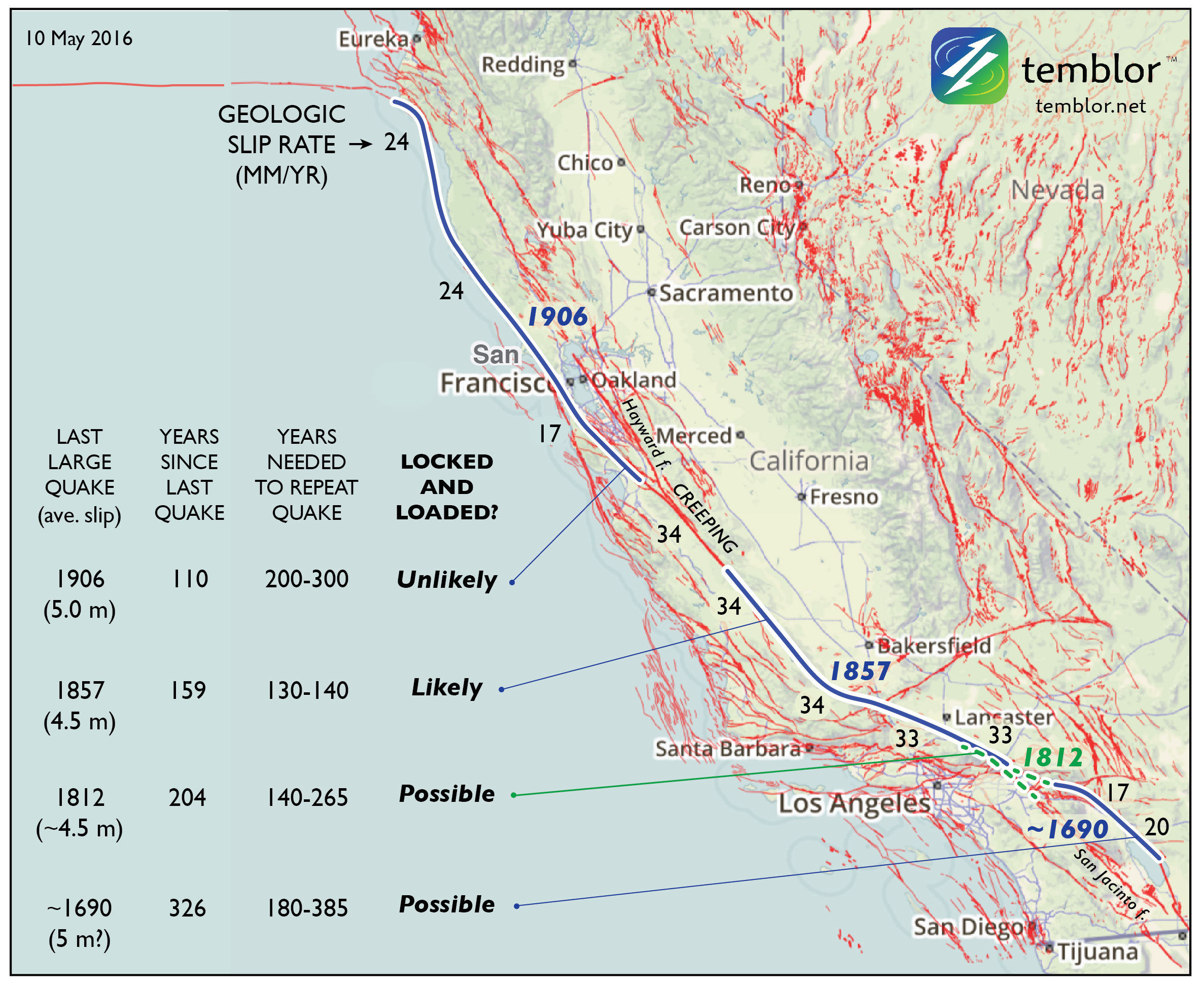
So, how about ‘locked, generally loaded, with some sections perhaps ready to go’
When I repeat Tom’s assessment in the accompanying map and table, I get a more nuanced answer. Even though the time since the last great quake along the southernmost San Andreas is longest, the slip rate there is lowest, and so this section may or may not have accumulated sufficient stress to rupture. And if it were ready to go, why didn’t it rupture in 2010, when the surface waves of the Mw=7.2 El Major-Cucapah quake just across the Mexican border enveloped and jostled that section? The strongest case can be made for a large quakeoverlapping the site of the Great 1857 Mw=7.8 Ft. Teton quake, largely because of the uniformly high San Andreas slip rate there. But this section undergoes a 40° bend (near the ‘1857’ in the map), which means that the stresses cannot be everywhere optimally aligned for failure: it is “locked” not just by friction but by geometry.
A reality check from Turkey
Sometimes simplicity is a tantalizing mirage, so it’s useful to look at the San Andreas’ twin sister in Turkey: the North Anatolian fault. Both right-lateral faults have about the same slip rate, length, straightness, and range of quake sizes; they both even have a creeping section near their midpoint. But the masterful work of Nicolas Ambraseys, who devoured contemporary historical accounts along the spice and trade routes of Anatolia to glean the record of great quakes (Nick could read 14 languages!) affords us a much longer look than we have of the San Andreas.
The idea that the duration of the open interval can foretell what will happen next loses its luster on the North Anatolian fault because it’s inter-event times, as well as the quake sizes and locations, are so variable. If this 50% variability applied to the San Andreas, no sections could be fairly described as ‘overdue’ today. Tom did not use this term, but others have. We should, then, reserve ‘overdue’ for an open interval more than twice the expected inter-event time.
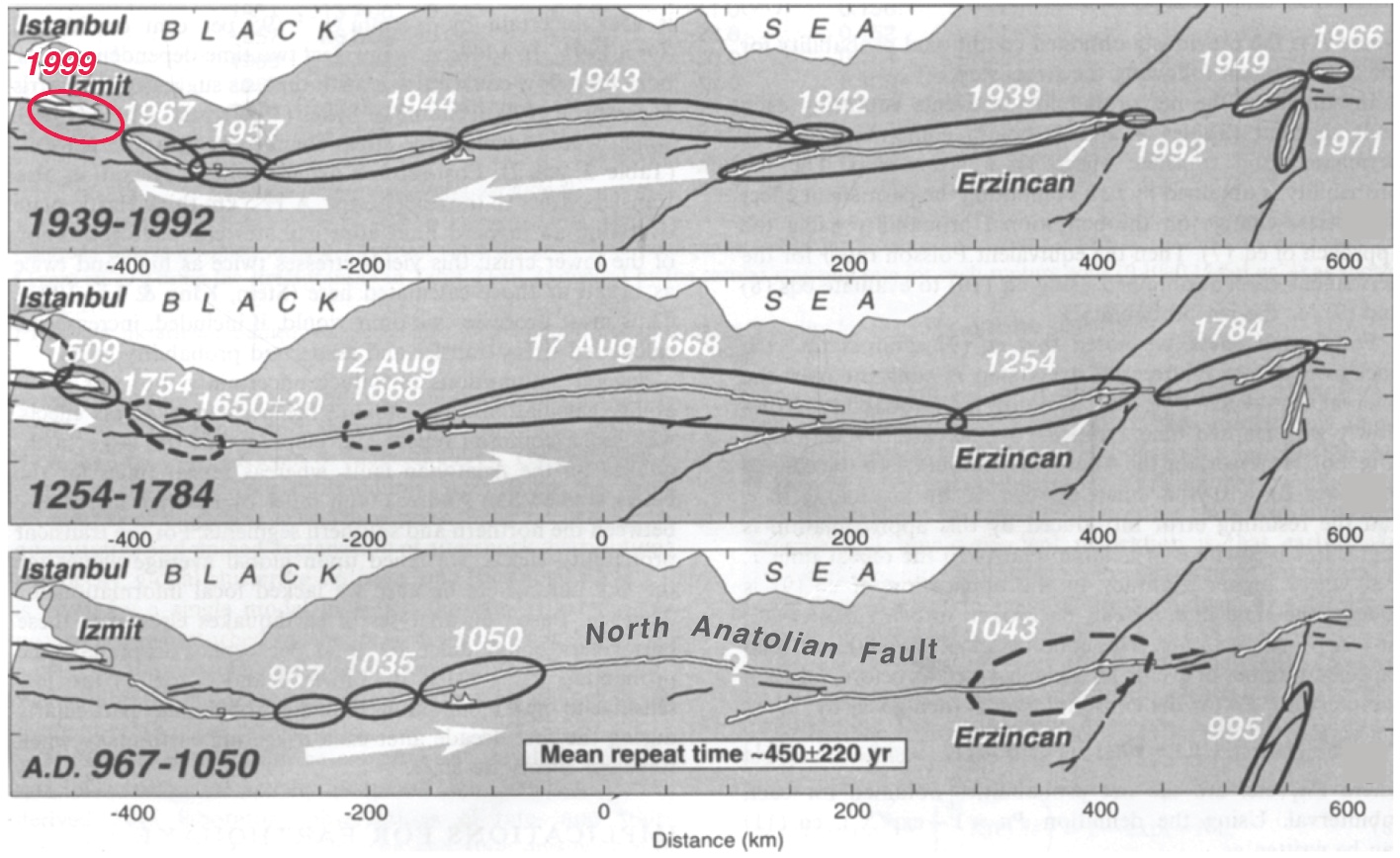
However, another San Andreas look-alike, the Alpine Fault in New Zealand, has a record of more regular earthquakes, with an inter-event variability of 33% for the past 24 prehistoric quakes (Berryman et al., 2012). But the Alpine fault is straighter and more isolated than the San Andreas and North Anatolian faults, and so earthquakes on adjacent faults do not add or subtract stress from it. And even though the 31 mm/yr slip rate on the southern Alpine Fault is similar to the San Andreas, the mean inter-event time on the Alpine is longer than any of the San Andreas’ open intervals: 330 years. So, while it’s fascinating that there is a ‘metronome fault’ out there, the Alpine is probably not a good guidepost for the San Andreas.
If Tom’s slide is too simple, and mine is too equivocal, what’s the right answer?
I believe the best available answer is furnished by the latest California rupture model, UCERF3. Rather than looking only at the four San Andreas events, the team created hundreds of thousands of physically plausible ruptures on all 2,000 or so known faults. They found that the mean time between Mw≥7.7 shocks in California is about 106 years (they report an annual frequency of 9.4 x 10^-3 in Table 13 of Field et al., 2014; Mw=7.7 is about the size of the 1906 quake; 1857 was probably a Mw=7.8, and 1812 was probably Mw=7.5). In fact, this 106-year interval might even be the origin of Tom’s ‘once per century’ expectation since he is a UCERF3 author.
But these large events need not strike on the San Andreas, let alone on specific San Andreas sections, and there are a dozen faults capable of firing off quakes of this size in the state. While the probability is higher on the San Andreas than off, in 1872 we had a Mw=7.5-7.7 on the Owen’s Valley fault (Beanland and Clark, 1994). In the 200 years of historic records, the state has experienced up to three Mw≥7.7 events, in southern (1857) and eastern (1872), and northern (1906) California. This rate is consistent with, or perhaps even a little higher than, the long-term model average.
So, what’s the message
While the southern San Andreas is a likely candidate for the next great quake, ‘overdue’ would be over-reach, and there are many other fault sections that could rupture. But since the mean time between Mw≥7.7 California shocks is about 106 years, and we are 110 years downstream from the last one, we should all be prepared—even if we cannot be forewarned.
Ross Stein (ross@temblor.net), Temblor
You can check your home’s seismic risk at Temblor
References cited:
Sarah Beanland and Malcolm M. Clark (1994), The Owens Valley fault zone, eastern California, and surface faulting associated with the 1872 earthquake, U.S. Geol. Surv. Bulletin 1982, 29 p.
Kelvin R. Berryman, Ursula A. Cochran, Kate J. Clark, Glenn P. Biasi, Robert M. Langridge, Pilar Villamor (2012), Major Earthquakes Occur Regularly on an Isolated Plate Boundary Fault, Science, 336, 1690-1693, DOI: 10.1126/science.1218959
James H. Dietrich and Keith Richards-Dinger (2010), Earthquake recurrence in simulated fault systems, Pure Appl. Geophysics, 167, 1087-1104, DOI: 10.1007/s00024-010-0094-0.
Edward H. (Ned) Field, R. J. Arrowsmith, G. P. Biasi, P. Bird, T. E. Dawson, K. R., Felzer, D. D. Jackson, J. M. Johnson, T. H. Jordan, C. Madden, et al.(2014). Uniform California earthquake rupture forecast, version 3 (UCERF3)—The time-independent model, Bull. Seismol. Soc. Am. 104, 1122–1180, doi: 10.1785/0120130164.
Robert Graves, Thomas H. Jordan, Scott Callaghan, Ewa Deelman, Edward Field, Gideon Juve, Carl Kesselman, Philip Maechling, Gaurang Mehta, Kevin Milner, David Okaya, Patrick Small, Karan Vahi (2011), CyberShake: A Physics-Based Seismic Hazard Model for Southern California, Pure Appl. Geophysics, 168, 367-381, DOI: 10.1007/s00024-010-0161-6.
Julian C. Lozos (2016), A case for historical joint rupture of the San Andreas and San Jacinto faults, Science Advances, 2, doi: 10.1126/sciadv.1500621.
Tom Parsons, K. M. Johnson, P. Bird, J.M. Bormann, T.E. Dawson, E.H. Field, W.C. Hammond, T.A. Herring, R. McCarey, Z.-K. Shen, W.R. Thatcher, R.J. Weldon II, and Y. Zeng, Appendix C—Deformation models for UCERF3, USGS Open-File Rep. 2013–1165, 66 pp.
Seok Goo Song, Gregory C. Beroza and Paul Segall (2008), A Unified Source Model for the 1906 San Francisco Earthquake, Bull. Seismol. Soc. Amer., 98, 823-831, doi: 10.1785/0120060402
Kerry E. Sieh (1978), Slip along the San Andreas fault associated with the great 1857 earthquake, Bull. Seismol. Soc. Am., 68, 1421-1448.
Ross S. Stein, Aykut A. Barka, and James H. Dieterich (1997), Progressive failure on the North Anatolian fault since 1939 by earthquake stress triggering, Geophys. J. Int., 128, 594-604, 1997, 10.1111/j.1365-246X.1997.tb05321.x
Simpson Strong-Tie® Strong-Wall® Wood Shearwall – The Latest in Our Prefabricated Shearwall Panel Line Part 1
Some contractors and framers have large hands, which can pose a challenge for them when they’re trying to install the holdown nuts used to attach our Strong-Wall® SB (SWSB) Shearwall product to the foundation. Couple that challenge with the fact that anchorage attachment can only be achieved from the edges of the SWSB panel, and variable site-built framing conditions can limit access depending upon the installation sequence. To alleviate anchorage accessibility issues, we’ve required a gap between the existing adjacent framing and SWSB panel equal to the width of a 2x stud to provide access so the holdown nut can be tightened. Even so, try telling a framer an inch and a half is plenty of room in which to install the nut!Continue Reading
Habitat STRONG Blog
This week’s post was written by Kevin Gobble of Habitat for Humanity. Kevin is the Program Manager for Habitat for Humanity’s new Habitat Strong initiative. Kevin has spent over 22 years in residential construction building energy-efficient, high-performing home, and has consulted with several sustainable building programs on ways to develop their own best practices. As a third-generation builder, he has knowledge in the field of residential building science and has furthered his education to include many industry certifications — NARI Certified Remodeler, NAHB Certified Green Professional, RESNET Certified Green Rater, BPI Building Analyst, FORTIFIED evaluator, and Level 1 Infrared Thermography — while working directly with industry partners to focus on cost-effective construction solutions. Kevin has built and remodeled numerous homes to high-performance standards as certified by various building programs, including his latest project for himself: converting a condemned historic property in Atlanta to EarthCraft House Platinum.
In a previous blog post, we discussed the background of the Habitat Strong program. Habitat Strong promotes the building of resilient homes that are better equipped to withstand natural disasters in every region of the country. This program uses IBHS FORTIFIED Home™ standards and works well within Habitat’s model of building affordable, volunteer-friendly homes.Continue Reading